Key Takeaways
1. Rocketry's Birth: The Essential Need for Liquid Propellants
Space travel is possible. This can be accomplished by means of, and only by means of, rocket propulsion, since a rocket is the only known propulsive device which will work in empty space.
Early visionaries. The dream of space travel, articulated by Konstantin Tsiolkovsky in 1903, hinged entirely on rocket propulsion. He correctly identified that only a rocket, carrying both fuel and oxidizer, could function in the vacuum of space, unlike air-breathing engines. This fundamental insight shifted focus from gunpowder rockets, which lacked sufficient energy, to the potential of liquid propellants.
Identifying key liquids. Tsiolkovsky's theoretical work pinpointed specific liquids with the necessary energy density for spaceflight. His most notable suggestion was the combination of liquid hydrogen as a fuel and liquid oxygen as an oxidizer. This was a revolutionary idea, only possible because scientists like Wroblewski, Olszewski, and Dewar had recently succeeded in liquefying these "permanent gases" in the late 19th century.
The dawn of possibility. The liquefaction of oxygen (1883) and hydrogen (1898) provided the physical reality needed for Tsiolkovsky's theoretical rockets. Without these cryogenic breakthroughs, the concept of liquid-fueled space travel would have remained purely speculative. This early period established the critical link between advanced chemistry and the nascent field of rocketry.
2. Early Pioneers Laid the Foundation for Liquid Propulsion
By November 1923 he had fired a rocket motor on the test stand, and on March 16, 1926, he achieved the first flight of a liquid-propelled rocket.
Goddard's practical steps. While Tsiolkovsky theorized, Robert H. Goddard in the United States began experimental work. Unaware of Tsiolkovsky's writings, Goddard independently concluded that liquid hydrogen and oxygen were ideal but, facing the impracticality of liquid hydrogen, chose gasoline and liquid oxygen for his experiments. His persistent efforts led to the first liquid-fueled rocket flight in 1926.
Diverse early explorations. The 1920s and 30s saw independent groups emerge globally, often in secrecy.
- Russia: Tsander and Glushko experimented with gasoline/LOX and toluene/nitrogen tetroxide (N2O4).
- Italy: Luigi Crocco pioneered the use of N2O4 with gasoline, a significant step towards storable oxidizers, though his work remained unknown for decades.
- Germany: The VfR (Society for Space Travel) worked with alcohol/LOX, leading directly to the V-2. Friedrich Sander introduced red fuming nitric acid (RFNA) as an oxidizer.
- France: Esnault-Pelterie explored benzene/N2O4 and disastrously, tetranitromethane.
Monopropellant concepts emerge. Alongside bipropellants (fuel + oxidizer), the idea of monopropellants (fuel and oxidizer in one liquid) was explored. Luigi Crocco bravely experimented with nitroglycerine and nitromethane, while Helmuth Walter developed motors using high-strength hydrogen peroxide, a monopropellant that decomposes into hot gas. These early, often dangerous, trials laid the groundwork for future propellant classes.
3. The Crucial Quest for Storable Propellants Began in Wartime
When you get word from your forward observers that the bombers are on the way, you don’t have time to load up a missile with liquid oxygen.
Military necessity drives change. World War II shifted rocketry from theoretical exploration and sounding rockets to practical weapons. The need for anti-aircraft missiles demanded propellants that could be stored in the missile tanks, ready for instant firing, unlike cryogenic liquid oxygen which boils away. This military requirement spurred the development of "storable" propellants.
Germany's storable push. Germany, particularly through Helmuth Walter's work, focused on high-strength hydrogen peroxide ("T-Stoff") and fuels like hydrazine hydrate/methanol ("C-Stoff"). This led to rocket-powered aircraft like the Me 163. BMW and others also vigorously pursued nitric acid as a storable oxidizer, discovering that certain fuels ignited spontaneously upon contact – a critical property they termed "hypergolic."
US follows suit. In the US, GALCIT (later JPL) and Reaction Motors (RMI) were tasked with developing JATO (Jet-Assisted Take-Off) units for aircraft. The Army Air Corps specifically demanded storable oxidizers. JPL initially struggled with RFNA and gasoline but, upon learning of the Navy's discovery, quickly adopted the aniline-RFNA combination, achieving the first US liquid JATO flight in 1942.
The storable challenge. The early storable propellants like aniline and RFNA were highly toxic, corrosive, and difficult to handle, freezing at inconvenient temperatures. This immediately launched a major research effort to find less noxious, more stable, and lower-freezing alternatives, setting the stage for intense post-war propellant development.
4. Nitric Acid: Taming the Corrosive Beast
Ha! If they had known the trouble that nitric acid was to cause before it was finally domesticated, the authors would probably have stepped out of the lab and shot themselves.
A necessary evil. Red Fuming Nitric Acid (RFNA) and White Fuming Nitric Acid (WFNA) were among the first widely used storable oxidizers due to their availability and energy. However, they were notoriously difficult to live with.
- Extreme corrosivity: Chewed through aluminum and stainless steel tanks, forming sludges that clogged injectors.
- Toxicity: Emitted dense, poisonous fumes (NO2) and caused severe burns on contact.
- Instability: WFNA decomposed spontaneously, building pressure in tanks.
The battle for domestication. A massive, multi-year effort involving numerous labs (JPL, NARTS, RMI, etc.) was waged to make nitric acid usable.
- Freezing point: Additives like ammonium nitrate were explored, but often degraded performance or stability.
- Decomposition: Studies mapped the complex HNO3-N2O4-H2O system, leading to "Stable Fuming Nitric Acid" (SFNA) with controlled N2O4/water content to reduce pressure buildup.
- Corrosion: A breakthrough at NARTS discovered that adding a small amount of hydrofluoric acid (HF) dramatically inhibited corrosion in aluminum and stainless steel, a trick later adopted globally.
A usable oxidizer emerges. By the mid-1950s, inhibited RFNA (IRFNA) was developed, significantly reducing corrosion and decomposition issues. This allowed missiles to be pre-packaged, eliminating hazardous field handling. Despite its remaining toxicity, IRFNA became a workhorse oxidizer for tactical missiles and some space applications, a testament to the chemists' perseverance in taming a truly nasty substance.
5. Hypergolicity: The Magic of Instant, Reliable Ignition
His code name for nitric acid was “Ignol” and for his fuels “Ergol,” and, a fast man with a Greek root, he came up with “Hypergol” for the spontaneous igniters.
Solving the ignition problem. Starting a rocket motor reliably is a major challenge. Early methods used spark plugs or pyrotechnics, which were often unreliable and added complexity. The discovery of hypergolicity – the spontaneous ignition of fuel and oxidizer upon contact – offered a much simpler and more dependable solution.
German origins. German researchers like Noeggerath and von Zborowski, working with nitric acid, systematically searched for fuels that would ignite instantly. They coined the term "hypergol" for these combinations. Early hypergolic fuels included aniline and turpentine, later leading to complex mixtures based on aromatic and tertiary amines ("Tonkas," "Ergols").
US adoption and search. The US quickly adopted the aniline-RFNA hypergolic pair for early JATOs after its discovery by Ensign Stiff. The search for better hypergols intensified post-war.
- Furfuryl alcohol: Found to be hypergolic with nitric acid and less toxic than aniline.
- Amines: Tertiary amines like triethylamine and polyamines were systematically investigated for their hypergolic properties.
- Sulfur compounds: Mercaptans and thiophosphites were found to be hypergolic but often had terrible odors and toxicity.
Screening methods developed. To avoid blowing up motors during the search, labs developed ignition delay testers, dropping small amounts of propellants together to measure the time to ignition. While results varied between labs, these testers were crucial for screening thousands of potential hypergolic combinations, guiding the development towards safer and more reliable propellant pairs.
6. Hydrazines: The Storable Fuel Family Triumphs Over Challenges
The substituted hydrazine program was a resounding success. It had made all the other storable fuels completely obsolete.
The ideal storable fuel candidate. Hydrazine (N2H4) was recognized early on as a highly promising storable fuel due to its high energy, good density, and hypergolicity with key oxidizers like nitric acid and N2O4. However, pure hydrazine had a major drawback: a freezing point just below water (1.5°C), far too high for military use in varied climates.
The quest for low freezing points. Extensive efforts were made to lower hydrazine's freezing point without sacrificing performance or stability.
- Water/Ammonia: Lowered freezing point but significantly degraded performance and density.
- Methanol: Improved freezing point but reduced performance.
- Hydrazine nitrate: Lowered freezing point but created dangerously shock-sensitive mixtures.
- Lithium borohydride: Showed promise but mixtures were unstable.
The substituted hydrazine breakthrough. The key came from synthesizing hydrazine derivatives. Monomethylhydrazine (MMH) and unsymmetrical dimethylhydrazine (UDMH) were found to have significantly lower freezing points (-52.4°C and -57.2°C respectively) while retaining high performance and hypergolicity. A mixture of UDMH and MMH froze even lower (-80°C).
Dominance in storable fuels. UDMH and MMH, alone or in mixtures (like Aerozine 50, a 50/50 UDMH/Hydrazine blend), became the standard storable fuels. They offered excellent performance, reliable hypergolic ignition, and acceptable handling properties compared to earlier fuels. This family of compounds largely ended the search for new storable fuels, becoming the "workhorse" fuels for tactical and strategic missiles using nitric acid or N2O4.
7. Peroxide: The Promising Oxidizer That Never Quite Made It
Peroxide just didn’t make it.
Initial appeal. High-strength hydrogen peroxide (H2O2) held significant promise as a storable oxidizer. It was less toxic and corrosive than nitric acid and offered comparable performance with many fuels. It could also be used as a monopropellant, decomposing to hot gas for auxiliary power.
Major drawbacks. Despite its advantages, peroxide faced critical challenges that ultimately limited its widespread adoption as a main oxidizer:
- High freezing point: Pure H2O2 freezes near 0°C. While lower concentrations or additives like ammonium nitrate helped, they often reduced performance or increased instability.
- Instability: H2O2 decomposition is exothermic and self-accelerating, easily catalyzed by trace contaminants (metals, dirt, organics). Maintaining the required "levitical" cleanliness was extremely difficult in practice.
- Handling risks: Spills on organic materials could cause fires. Mixing with fuels like jet fuel could lead to violent detonations under certain conditions, a risk deemed unacceptable for shipboard use.
Limited applications. While peroxide found success as a monopropellant for auxiliary power (like in the V-2's turbopumps) and torpedoes, its instability and handling risks prevented it from becoming a primary oxidizer for missiles, especially compared to the successfully domesticated nitric acid and N2O4. Despite continued research, it remained "always a bridesmaid."
8. Halogens: Powerful, Perilous, and Promising for Performance
It is also quite probably the most vigorous fluorinating agent in existence—much more vigorous than fluorine itself.
Seeking higher performance. Beyond oxygen-based oxidizers, fluorine and its compounds offered the potential for significantly higher performance due to the strong bonds formed in combustion products like HF. The search for storable halogen oxidizers focused on interhalogens like chlorine trifluoride (ClF3).
ClF3: Power and peril. ClF3 ("CTF") offered excellent performance and hypergolicity with virtually all fuels. However, its extreme reactivity made it incredibly dangerous to handle.
- Reactivity: Ignited spontaneously with most materials, including water, sand, and even some metals if the protective fluoride layer was breached.
- Handling: Required specialized, meticulously cleaned metal plumbing (steel, copper, aluminum) and presented risks of metal-fluorine fires.
- Motor issues: High combustion temperatures (near 4000K) were extremely hard on injectors and nozzles.
Other halogen oxidizers. Bromine pentafluoride (BrF5) was similar but harder to get good performance from. Perchloryl fluoride (FClO3) was much safer to handle but had lower density and a high expansion coefficient. Nitrogen fluorides like N2F4 offered high performance but low boiling points, leading to the concept of "space-storable" propellants. ONF3 was later discovered, offering better stability than N2F4.
Future role. While handling difficulties and cost limited their widespread use, halogen oxidizers, particularly ClF5 and OF2 (as a cryogenic/space-storable), remain attractive for applications demanding maximum performance, such as deep space missions, where their challenges are more manageable.
9. Boranes: High Hopes, High Costs, and High Toxicity
Actually, the boron programs did not cost a billion dollars. It just seemed that way at the time.
The allure of high energy. Boranes (compounds of boron and hydrogen) possessed exceptionally high heats of combustion, sparking intense interest as potential high-energy fuels for both jet engines and rockets starting in the 1950s. Pentaborane (B5H9) and diborane (B2H6) were key candidates.
Project Zip: A costly endeavor. The US Navy and Air Force launched multi-million dollar programs ("Zip," "HEF") to develop boron-based jet fuels. This led to large-scale synthesis and production facilities for alkyl boranes. However, the program ultimately failed because the combustion product, boron trioxide (B2O3), formed sticky glass deposits that destroyed turbine engines.
Rocket applications limited. Despite the failure in jet engines, the availability of boranes spurred rocket research.
- Early firings: Showed poor performance and nozzle clogging with B2O3 deposits when burned with oxygen or peroxide.
- BN system: The concept of reacting boranes with hydrazine to form solid boron nitride (BN) and hydrogen gas offered high theoretical performance and lower temperatures, but struggled with combustion efficiency and injector design.
- Handling: Boranes are highly toxic, spontaneously flammable in air, and difficult to extinguish, posing significant safety challenges.
Legacy of the boranes. The massive investment in boranes did advance boron chemistry significantly, leading to discoveries like the stable carboranes. However, for liquid rocket propulsion, the practical challenges of combustion efficiency (especially with oxygen-type oxidizers), toxicity, cost, and handling largely outweighed the performance benefits, rendering many borane systems obsolete upon development.
10. Monopropellants: Simple Concept, Explosive Reality
Any intimate mixture of a fuel and an oxidizer is a potential explosive, and a molecule with one reducing (fuel) end and one oxidizing end, separated by a pair of firmly crossed fingers, is an invitation to disaster.
The appeal of simplicity. Monopropellants, containing both fuel and oxidizer in a single liquid, promised simplified plumbing and mixture ratio control compared to bipropellants. They were explored for auxiliary power units (APUs) and main propulsion.
Low-energy monoprops. Hydrogen peroxide found success as an APU monopropellant. Propyl nitrate was also investigated but proved dangerously sensitive to adiabatic compression (detonating from rapid pressure changes in liquid lines), leading to its abandonment for most uses. Ethylene oxide was used for APUs but had coking and polymerization issues. Hydrazine became a widely used APU monopropellant, though reliable ignition and restarts remained challenging.
High-energy quest and dangers. The search for high-energy monopropellants to compete with bipropellants was fraught with peril.
- Nitromethane: Required additives for desensitization and catalysts for efficient combustion ("Neofuel"), but remained sensitive.
- Amine nitrates in nitric acid: A major research thrust, leading to compounds like "Isolde" and "Cavea." While offering good performance and improved stability (especially quaternaries like "Tallulah" and "Cavea B"), they still posed detonation risks, particularly in large quantities or under shock.
- Exotic mixtures: Attempts to mix unstable components like N2O4/hydrocarbons or N-F compounds/oxidizers often resulted in violent explosions.
Ultimately limited. Despite significant effort and chemical ingenuity, high-energy monopropellants struggled to balance performance with safety and stability requirements for operational use. The inherent risk of having fuel and oxidizer intimately mixed proved too difficult to overcome for main propulsion applications, though low-energy monoprops like hydrazine remain valuable for auxiliary tasks.
11. The Human Element: Personalities, Politics, and Progress
Conformists were hard to find in the group. Almost to a man, they were howling individualists.
A community of characters. The world of liquid propellant development was populated by a small, tight-knit group of highly individualistic chemists and engineers. They were intellectual rivals, fiercely competitive, yet relied on each other for informal information exchange and validation of their work, often meeting at bars during conferences.
Bureaucracy and funding. Research was heavily dependent on military funding (Army, Navy, Air Force, ARPA). Funding priorities shifted based on strategic needs (bombers vs. missiles), political pressures, and sometimes, seemingly arbitrary decisions or security breaches. Projects could be canceled abruptly, and inter-service rivalries influenced propellant choices (e.g., the Navy's initial aversion to nitric acid on carriers).
Accidents and lessons learned. The dangerous nature of the work meant accidents were inevitable, though surprisingly few
[ERROR: Incomplete response]
Last updated:
Review Summary
Ignition! is a highly entertaining and informative book about the history of rocket propellants. Readers praise Clark's witty writing style and fascinating anecdotes from the early days of rocket science. The book offers a unique blend of chemistry, history, and humor, making complex topics accessible. While some find the technical details challenging, most appreciate the insider's perspective on this dangerous field. The book is particularly enjoyable for those with a background in chemistry or rocket engineering, but even general readers find it engaging despite occasional dense passages.
Similar Books
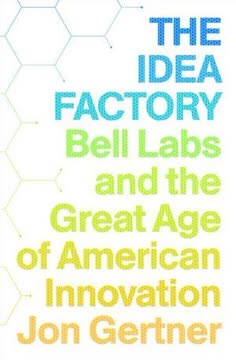
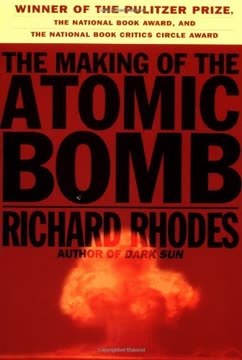
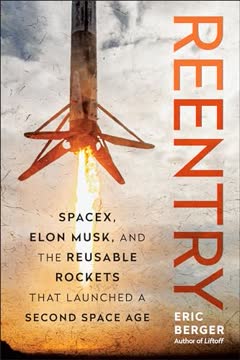
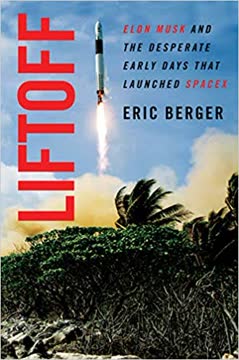
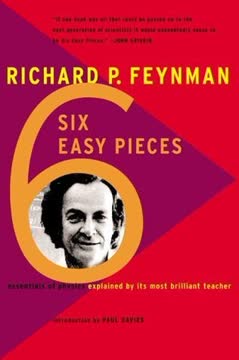
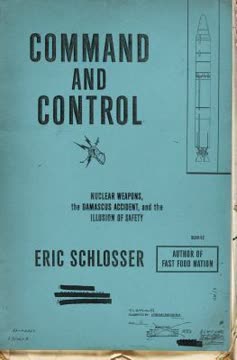